Research
Spatial Regulation of Cytoskeletal Dynamics During Tubular Organ Formation
Apical constriction is crucial for tissue invagination
In formation of tubular organs, one mechanism that forms a tube is budding of cells from an already polarized epithelial sheet: a subset of cells bud out of the plane of the epithelium to become the end of a new tube (Fig. 1). Tissue invagination by budding is observed in a simple epithelial tube such as Drosophila embryonic salivary gland (SG) as well as during branching morphogenesis of more complex organs, including the mammalian lungs and kidney. Correct tissue invagination is critical for making a well-formed tubular organ as defects at this initial step of tube formation could affect the entire tube shape or position.
A crucial cell shape change during tissue invagination is apical constriction, a widespread cellular process where the apical side of the cell becomes smaller than the other sides (Fig. 2). During tissue invagination, apical constriction creates cells with a wedge-like shape that can fit together to form the curved wall of the tube, similar to shaped bricks in an archway. Defective apical constriction causes failure or disruption of formation of many tubular organs.
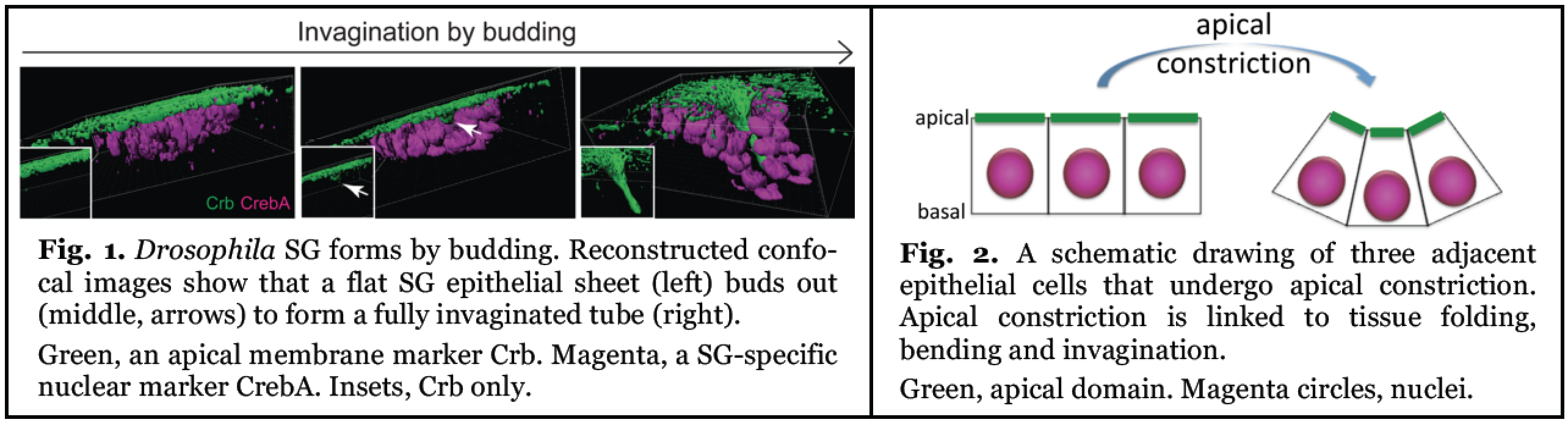
Fig. 1. Drosphilia SG forms by budding. Reconstructed confocal images show that a flat SG epithelial sheet (left) buds out (middle, arrows) to form a fully invaginated tube (right). Green, an apical membrane marker Crb. Magenta, a SG-specific nuclear marker CrebA. Insets, Crb only.
Fig. 2. A schematic drawing of three adjacent epithelial cells that undergo apical constriction. Apical constriction is linked to tissure folding, bending and invagination. Green, apical domain. Magenta circles, nuclei.
Contractile apical myosin drives apical constriction
The critical role of apical constriction in tube formation has been recognized for decades in both invertebrates and vertebrates. However, we only recently began to understand the molecular mechanisms of apical constriction, in large part from studies using Drosophila. This has revealed a novel and unexpected role for a distinct myosin structure created in response to cell-cell signaling and regulated by apical Rho-associated kinase (Rok). This myosin structure, which forms in the center of the apical end of cells, is highly pulsatile and generates the pulling force to drive apical constriction (hereafter referred to as apicomedial myosin; Fig. 3).
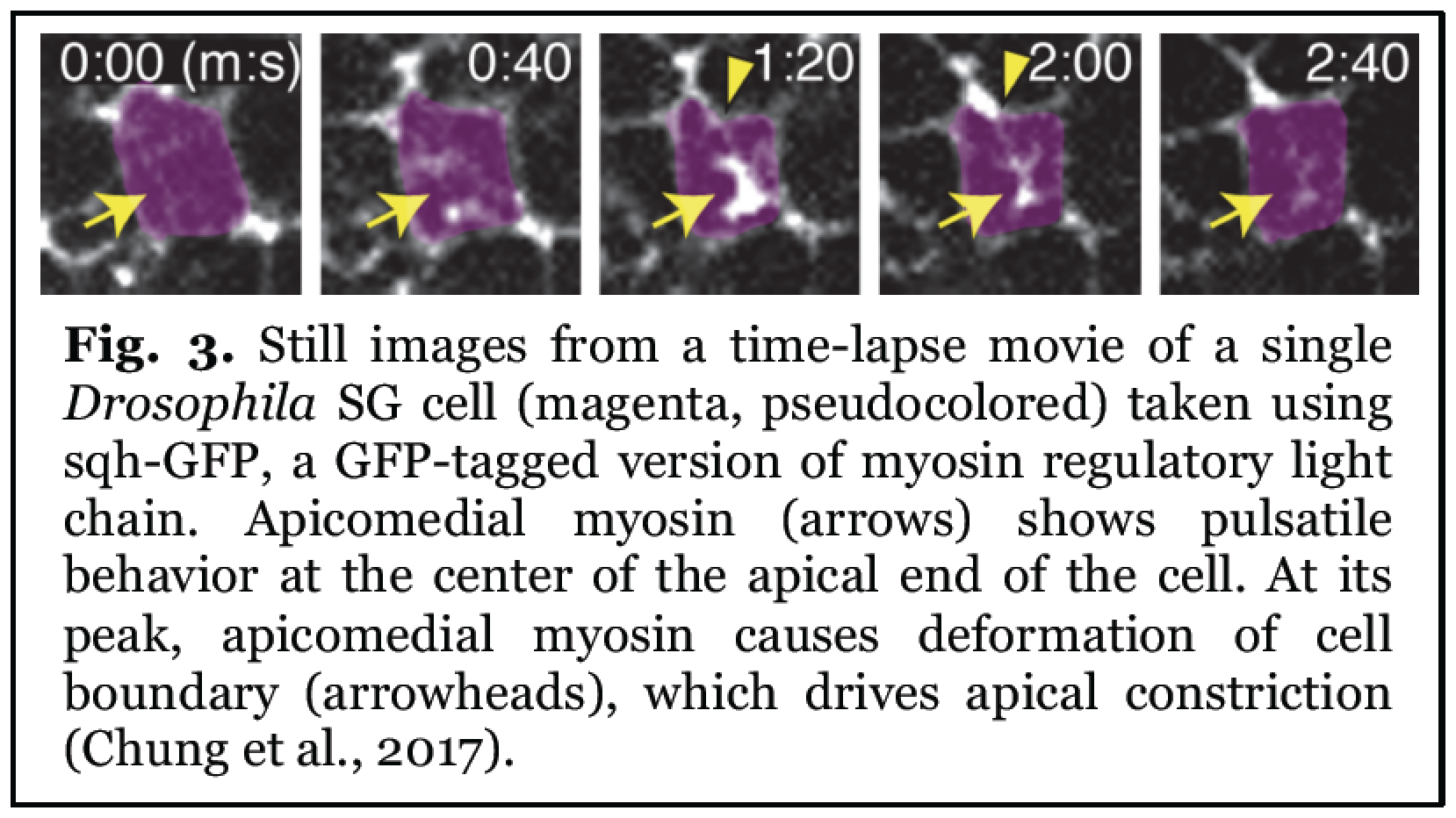
Still images from a time-lapse movie of a single Drosophila SG cell (magenta, pseudocolored) taken using sqh-GFP, a GFP-tagged version of myosin regulatory light chain. Apicomedial myosin (arrows) shows pulsatile peak, apicomedial myosin causes deformation of cell boundary (arrowheads), which drives apical constriction. - Chung et al., 2017
Drosophila SG is an excellent system to study apical constriction during tissue invagination
We use Drosophila embryonic SG as a model system to reveal the regulatory mechanisms of apical constriction during tissue invagination (Fig. 4). During Drosophila SG invagination, a group of cells first undergo apical constriction in the position of the future invagination site, and cells near the invagination site continue to constrict before they invaginate. When the SG begins to form, apical constriction appears to help the tissue bend and creates a “dimple” at the invagination site to start tube formation.
Our previous studies discovered a pathway that drives apical constriction during SG invagination: the SG transcription factor Fork head regulates expression of the secreted ligand Folded gastrulation (Fog), which promotes accumulation of Rok and apicomedial myosin formation (Fig. 4). We showed that Fog signaling activity is spatially biased: apically constricting cells are clustered at and near the invagination site (Fig. 4). We further showed that disruption of Fog signaling (e.g. fog mutants) results in abnormal tube formation, suggesting that localized actomyosin activity and the spatial coordination of apical constriction is critical for normal tube formation (Chung et al., 2017). We are currently working on understanding the mechanisms of how Fog signaling is regulated in a spatially controlled manner to drive apical constriction in cells clustered at a localized area.
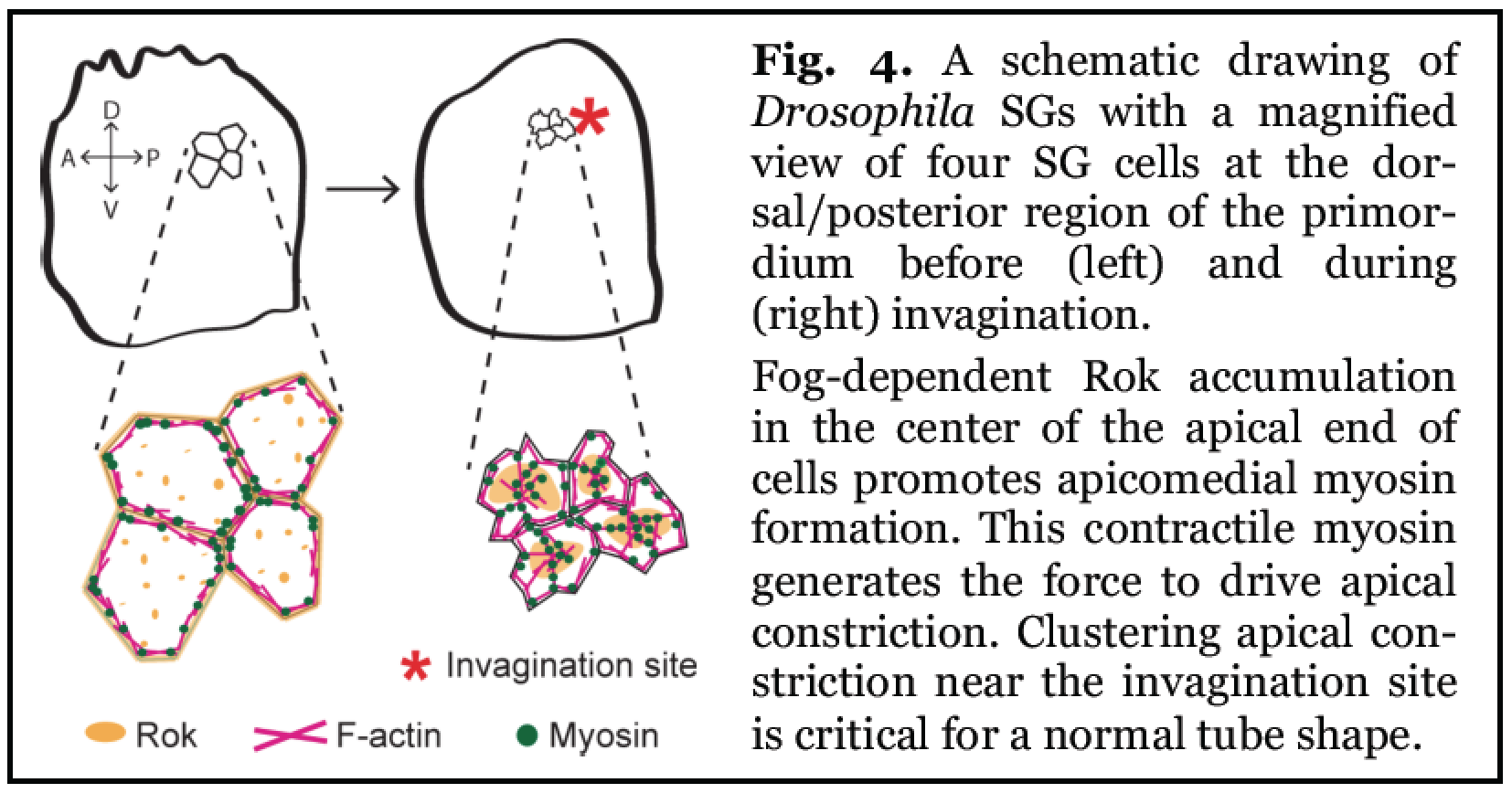
Fig. 4. A schematic drawing of Drosophila SGs with a magnified view of four SG cells at the dorsal/posterior region of the primordium before (left) and during (right) invagination.
Fog-dependent Rok accumulation in the center of the apical end of cells promotes apicomedial myosin formation. This contractile myosin generates the force to drive apical constriction. CLustering apical constriction near the invagination site is crirical for a normal tube shape.