Home
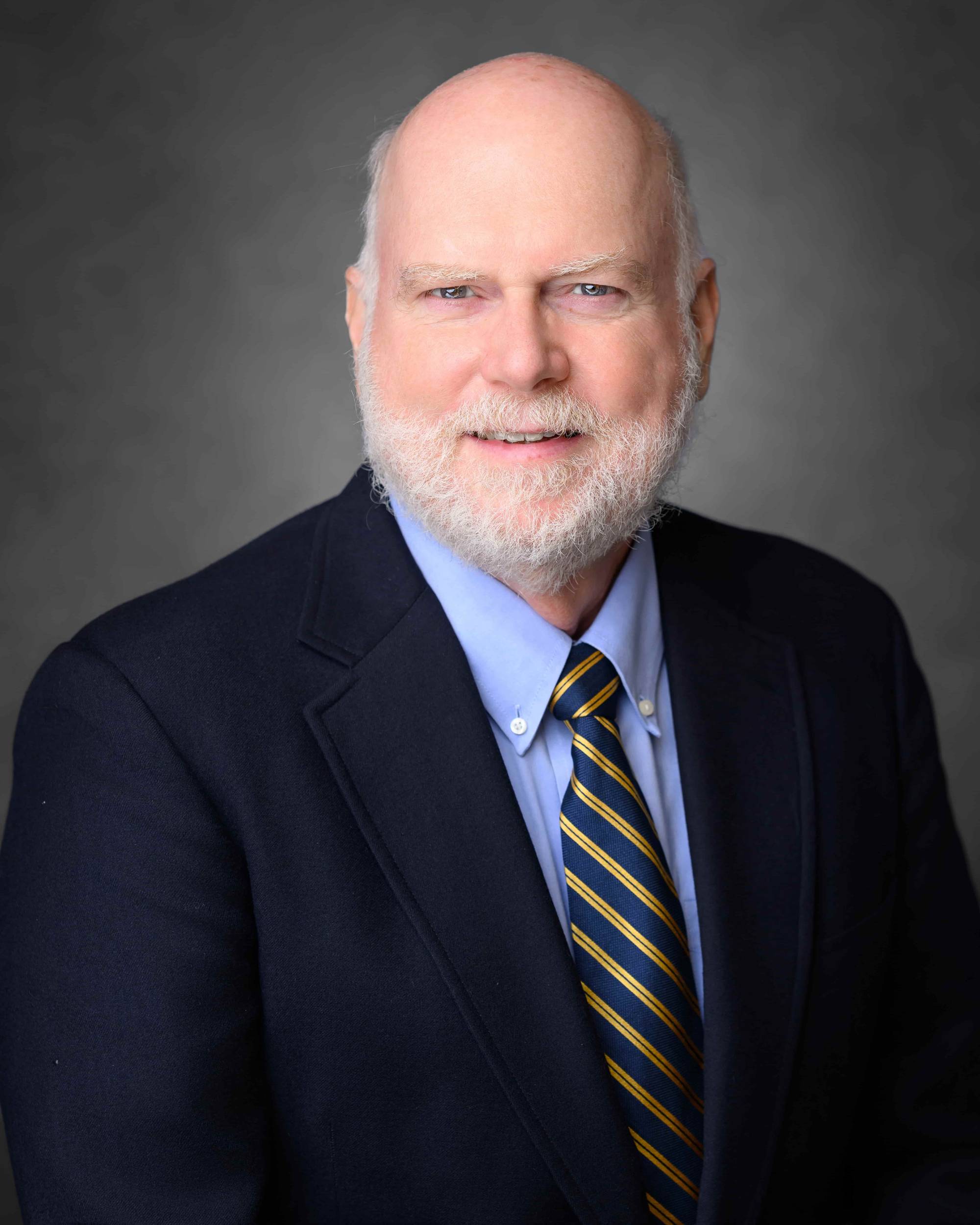
Les Butler
Professor – Inorganic and Materials Science
BS: University of Arkansas, 1977
PhD: University of Illinois at Urbana-Champaign, 1981
PostDoc: Caltech, 1981-83
Tomography and Interferometry
We are interested in developing new X-ray and neutron tomography/interferometry methods for applications in the chemical and materials sciences. In complex structures—batteries, 3D prints, anatomy—a 4D or 5D image is informative. Here, 3D is the spatial dimension and the additional dimensions are time, composition, or scattering. We have imaged the distribution of flame retardants in polymers [1], the uptake of hydrogen into LaNi5 alloy [2], and the neutron scattering due to residual porosity in 3D printed objects [3]. In comparison to previous imaging methods that only produce traditional attenuation (neutron) or absorption (X-ray) images, interferometry provides an additional two datasets: differential phase contrast (DPC) and dark-field. The dark-field signal can be correlated to the small angle scattering generated within a sample on the order of tens of nanometers to several micrometers. Hence, the main advantage of interferometry imaging is the ability to image large samples (mm to cm) while observing small features (nm to um). Here are a few the recent projects.
First Application of Talbot-Lau and Far-Field Neutron Interferometry for TiAl6V4 and SS316 Additive Manufacturing Test Objects
The possibility exists for one imaging method, such as attenuation, to measure defects in additively manufactured (AM) samples undetected with another imaging modality. Due to the chance of porosity defects occurring at multiple length scales (nanometer and micrometer), the need exists for performing high-resolution attenuation imaging and grating-based interferometry. The interferometry could detect changes in the small angle scattering (dark-field) while high-resolution imaging could magnify noticeable features through attenuation. For a several cm thick AM Ti-6Al-4V electron beam melted (EBM) sample, bulk properties regarding the shape, size and location of porosity defects were detected on the micron scale (1.97um) where a chimney-like pore structure was discovered [3]. There were two possibilities for the scattering differences: small gas-filled pockets present in the sample are less than 2 m or micron-scale phase separation of aluminum and titanium occurs in the material. If phase separation, the reduced neutron attenuation in the chimney-like features suggests aluminum enrichment. This project was the first application of grating-based interferometry with AM samples and showed the possibilities for future AM imaging projects.
Based on the results of the Ti-6Al-4V project, alternative setups to the Talbot-Lau interferometer have been designed for AM observation. Dr. Dan Hussey at the NIST Neutron Imaging Facility, working with Dr. Han Wen (NIH Heart, Blood, and Lung), reconfigured the NIST CG-X beamline into the world's first far-field neutron interferometer [4]. In this new design, the inter-grating distance G1-G2 or the sample to detector distance can be shortened/lengthened to probe a wide range of scattering lengths (roughly 50nm to 4um). In concern to AM samples, the stainless steel alloy 316 (SS316) is commonly used due to its properties of good ductility, a low deformation rate during cooling, and easy manipulation (bending or stretching). However, two problems in SS316 are tensile stress and fatigue, where thermal fatigue damage can be seen from a network of surface cracks propagating throughout a sample. Why is a chemist interested in broken stainless samples? Technique development! With tension-compression fatigue tests, it is possible to observe mechanical property changes, estimate fatigue damage in the microstructure, and determine the remaining lifetime of conventional and AM stainless steel dogbone samples. In combining the far-field interferometer with AM and conventional pristine, half-life, and fractured selective laser melted (SLM) SS316 dogbone samples that have undergone stress and fatigue testing, we were able to successfully determine the points of fracture using the dark-field imaging modality [5,6].
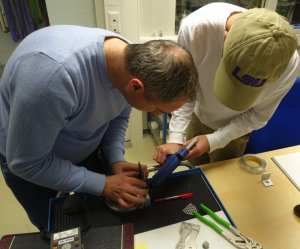
Fresh Data! This movie shows neutron tomography of stainless steel test samples using the Talbot-Lau interferometer; some are conventional and some are made with laser sintering additive manufacturing. Only 2D images of the samples were obtained using the far-field interferometer. The picture is of recent graduate Adam Brooks and our collaborator Nikolay Kardjilov fixing a sample stage for AM imaging. Our thanks for the Louisiana Consortium for Neutron Scattering, supported by the National Science Foundation EPSCoR and the Louisiana Board of Regents.
Since the samples above were too large for a tomography experiment with the far-field interferometer, a reduction in sample size was preferred. New samples for a tomography far-field interferometer experiment included 1cm SLM SS316 cubes and a quadratic. In this experiment, the inter-grating distance G1-G2 was increased such that scattering centers from 338nm up to 4.5um could be observed. Below is a movie showing four dark-field volumes at different scattering distances of the cubes and quadratic. This is the first known dark-field tomography experiment taken at different scattering lengths [7].
When looking at SS316 SLM cubes and a quadratic, the thought was that DPC and dark-field tomography could reveal internal features at different scattering lengths. While the DPC images were not valuable due to noise in the interferometer, the dark-field volumes did reveal changes in the dark-field signal by scanning different scattering lengths. On the right are volumes of the cubes and quadratic at scattering lengths of 338nm (top left), 675nm (top right), 1.01um (bottom left), and 1.35um (bottom right).
Flame Retardant Imaging
Our flame retardant/polymer 3D printing research needs a technique to measure the gaps between filament-filament layers in 3D printed objects. [1,8]
A picture of Adam Brooks and Les Butler working on the Keck interferometer instrument
in the Chemistry building. The interferometer operates similar to a rotisserie, where
the optical axis can be rotated 90 degrees to switch the gratings from a horizontal
to a vertical setup.
This science involves many collaborations. One example is the data processing of this "big data" problem. Prof. Warren Johnson, an LSU physicist from the LIGO (Laser Interferometer Gravitational-Wave Observatory) solved the math for fast, robust interferometry data processing. Who would have thought gravity wave research would impact 3D printing?
Other samples of interest are formanifera. The goal was to tell if this CaCO3 biological sample was uniform throughout the shell. On the right we observe an X-ray absorption volume of a formanifera and can see several regions of higher absorption.
Acknowledgements:
Our thanks to the W.M. Keck Foundation for funding this instrument construction. As best we can tell, it is one of six or so in the USA. It started working in January, 2017; the grant started in January, 2014. Actually, this is the second instrument. A prototype (turned permanent) was built at the LSU CAMD synchrotron and started working in May, 2015.
Here is a picture of our current research group at the ToScA North America Symposium in Austin, Texas (June 2017) with former LSU Vice Chancellor
of Research Lynn Jelinski and her husband. From left to right: Les Butler, Adam Brooks
(postdoc), Godfrey Mills (postdoc), Lynn Jelinski, Jumao Yuan (graduate student),
and Joe Jelinski. Not pictured: Omoefe Kio (graduate student)
A picture of our previous undergraduate and graduate students at the 2nd ICTMS conference
in Quebec City, Canada (June 2015). From left to right: Mutairu (Bolaji) Olatinwo
(graduated), Adam Brooks (postdoc), Omoefe (Joy) Kio (graduate student), and Gerald
(Gerry) Knapp (undergraduate). Bolaji currently works for the Intel Corporation, Adam
and Joy are at LSU, and Gerry has graduated and moved to the materials science program
at Penn State University.
March for Science
I marched in Washington, DC on April 22, 2017 to tell one story about chemistry. First
(no surprise), sometimes chemistry is hard. Fires are complicated chemical reactions.
For safety, a 3D printed component with embedded wiring should be protected with flame
retardants. Second hard problem: polymer blends are complicated. Adding a flame retardant
will affect the print quality. The neat thing about 3D printing is structure; it should
be possible to put the flame retardant exactly where it is needed, and not necessarily
on the surface. A fast method is needed for assessing 3D print quality as this and
that flame retardant is tested inside 3D printed objects. Third problem: how to look
at flame retardants and filament-filament gaps inside a 3D printed object? Our answer
is X-ray grating-based interferometry/tomography.
References:
- M. B. Olatinwo, K. Ham, J. McCarney, S. Marathe, J. Ge, G. Knapp, and L.G. Butler. Analysis of Flame Retardancy in Polymer Blends by Synchrotron X‑ray K‑edge Tomography and Interferometric Phase Contrast Movies. J. Phys. Chem. B, 2016, 120, 2612-2624.
- B. M. Wood, K. Ham, D. S. Hussey, D. L. Jacobson, A. Faradani, A. Kaestner, J. J. Vajo, P. Liu, T. A. Dobbens, and L. G. Butler. Real-Time Observation of Hydrogen Absorption by LaNi5 with Quasi-Dynamic Neutron Tomography:Establishing Time-Windows, Projection Weightings, and Image Processing. Nucl. Instrum. Methods B, 2013, 324, 95-101.
- A. J. Brooks, J. Ge, M. Kirka, R. Dehoff, H. Bilheux, N. Kardjilov, I. Manke, L.G. Butler. Porosity Detection in Electron Beam Melted Ti-6Al-4V using High-Resolution Neutron Imaging and Grating-Based Interferometry. Progress in Additive Manufacturing, 2017, 2(3), 125-132.
- D.S. Hussey, C. Brocker, J.C. Cook, D.L. Jacobson, T.R. Gentile, W.C. Chen, E. Baltic, D.V. Baxter, J. Doskow, M. Arif. A New Cold Neutron Imaging Instrument at NIST. Phys. Procedia, 2015, 69, 48–54.
- A. J. Brooks, D. S. Hussey, H. Yao, A. Haghshenas, J. Yuan, J. LaManna, D. L. Jacobson, C. G. Lowery, N. Kardjilov, S. Guo, M. M. Khonsari, L. G. Butler. Neutron Interferometry Detection of Early Crack Formation in Fatigued Additively Manufactured SS316 Dogbones. Materials & Design, 2018, 140, 420-430.
- A. J. Brooks, H. Yao, J. Yuan, O. Kio, C. G. Lowery, H. Markotter, N. Kardjilov, S. Guo, L. G. Butler. Early Detection of Fracture Failure in SLM AM Tension Testing with Talbot-Lau Neutron Interferometry. Additive Manufacturing, Under Review, 2017.
- A. J. Brooks, G. Knapp, J. Yuan, C. G. Lowery, M. Pan, B. E. Cadigan, S. Guo, D. S. Hussey, L. G. Butler. Neutron Imaging of Laser Melted SS316 Test Objects with Spatially Resolved Small Angle Neutron Scattering. J. Imaging, 2017, 3(4), 58.
- O. Kio, J. Yuan, A. J. Brooks, G. Knapp, K. Ham, J. Ge, D. van Loo, L. G. Butler. Non-Destructive Evaluation of Additively Manufactured Polymer Objects using X-ray Interferometry. Additive Manufacturing, Under Review Dec. 2017